
What limits solar cell efficiency?
Solar power is the conversion of energy from sunlight into electricity through the use of solar cells. Bell Laboratories in 1954 invented the silicon solar cell that became the eco-friendly future energy technology standard today. What defines solar power technology level is the conversion efficiency of a solar cell - the percentage of the solar energy that is converted into usable electricity. The higher the conversion efficiency, the more cost-competitive it is.
Both commercial and academic efforts have been focused on increasing the conversion efficiency of solar cells, resulting in the development of new materials and diverse solar cell structures for decades. As a result, conversion efficiency soared over the years. The most common type of solar cell used in households and industries are silicon-based solar cells and conversion efficiency reaches up to 27.6%. Theoretically, conversion efficiency for traditional single-junction solar cells can be increased by up to 34%.
The biggest obstacle to improving solar cell conversion efficiency is within the physical limitation that solar cells simply cannot absorb all of the light emitted by the sun. The sun emits wavelengths ranging from 250 to 2500 nm*, of which silicon-based solar cells absorb up to 500 to 1000 nm. Light with wavelengths longer than 1000nm passes through the solar cell, and light below 500 nm is absorbed but converted into heat - evaporating in the end.
Even if you were to utilize materials other than silicon to create solar cells, limits exist for conversion efficiency due to the fact that there are fixed wavelengths. This might make you wonder: Why is there a fixed wavelength in different materials? This can be explained by the fact that every resource has a specific energy band.
*Nanometer: One billionth of a meter
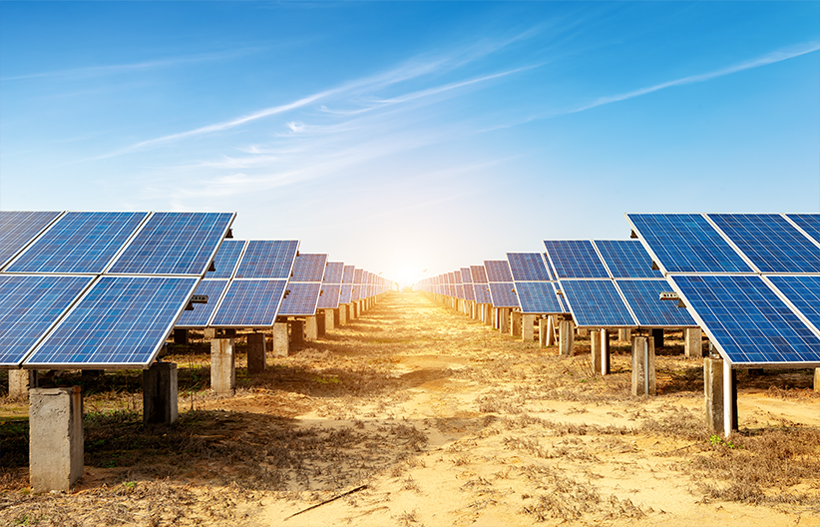
In the case of silicon-based solar cells, electrons within the silicon have to move to convert sunlight into electrical currents. However, electrons in silicon move only when the light at a wavelength shorter than 1000 nm is absorbed. Light at a wavelength of longer than 1000 nm does not have enough energy to move electrons inside silicon materials. In other words, light with a wavelength of more than 1000nm is useless for silicon solar cells.
On the other hand, a light at wavelengths below 500 nm has too much energy. Although it moves electrons inside silicon, the impact is so intense that heat is generated when electrons return to their original place. For silicon materials, light that can move electrons normally has wavelengths of between 500 and 1000 nm.
Due to these limitations, diversifying forms using polymeric organic materials or new materials such as perovskite or reducing production costs has become a priority for solar cells. A method of layering various types of solar cells as an effort to receive light from various wavelengths has also been developed, but this method is expensive to develop: It is only in use for high-tech equipment such as satellites.
Use of Quantum Dot Solar Cells on all Spectrum
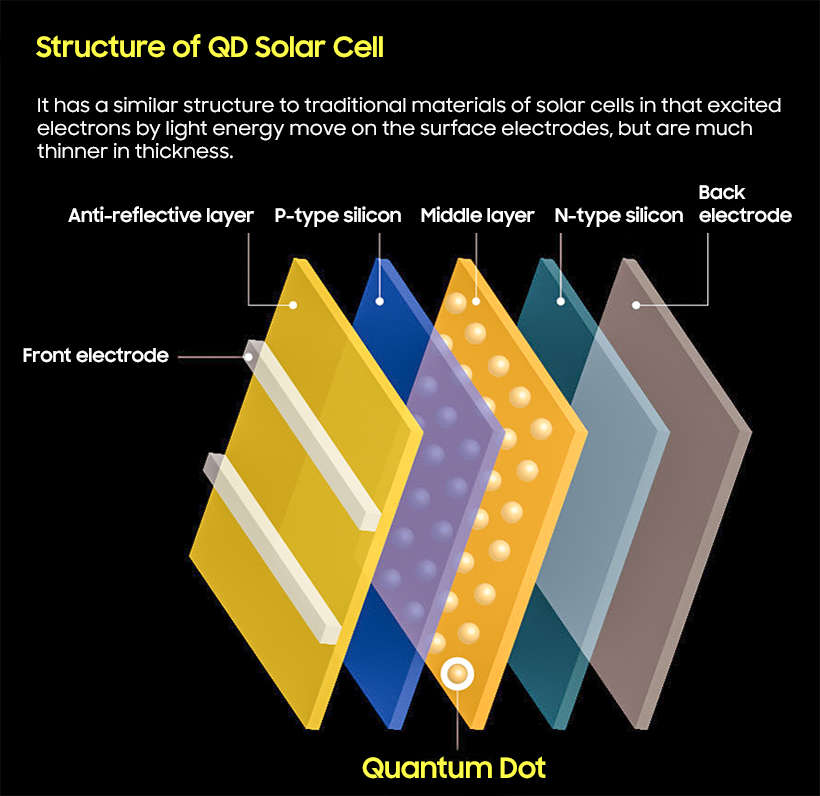
An astonishing study in solar cell development was published in 2004. Los Alamos National Laboratory under the U.S. Department of Energy observed that if a semiconductor particle, lead selenide (PbSe), is made as a nanometer, it does not generate heat when it receives short-wavelength light, but rather two or three times as many electrons are transported on the surface.
Currently Associate Professor of Department of Energy at Sungkyunkwan University (SKKU) in Korea, Sohee Jeong said, “This is a physical phenomenon called carrier multiplication. Utilizing quantum dot has the efficiency equivalent to that of a solar cell being powered by two suns.” She was a postdoctoral research associate studying quantum dots at Los Alamos National Lab.
When a material receives excessively high energy, if the material has an energy "band" it uses up the heat as the electron moves, but if the material has an energy "level" like a quantum dot, the story changes. When electrons move, heat does not occur, but rather power is multiplied by moving them to other electrons.
For example, if an electron in a quantum dot using lead selenide needs as much as 100 eV in order to move, two electrons move when 200 eV is given. In silicon materials, there is a big difference in energy production due to heat loss compared to when solar cells are made with quantum dots.
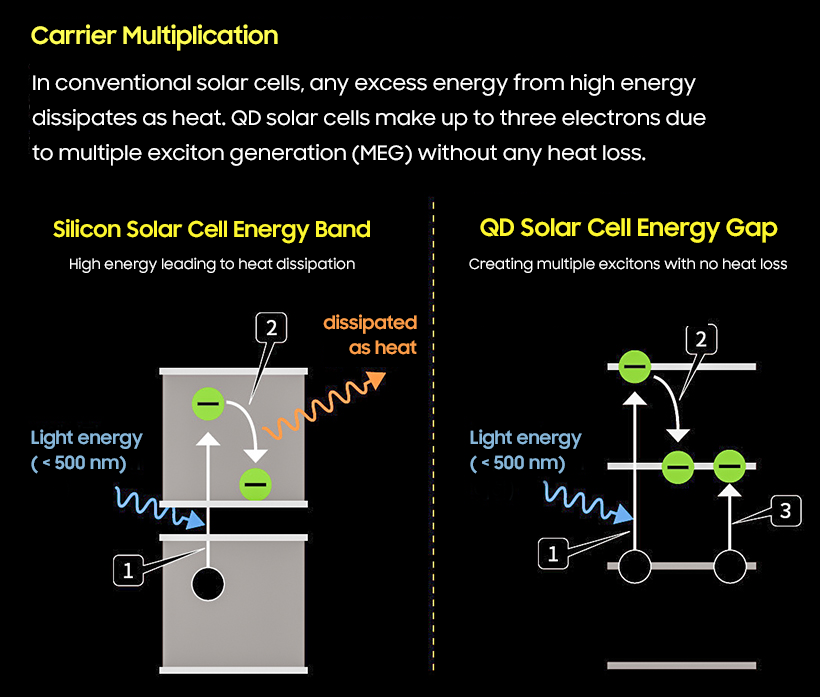
Professor Jeong said, "By adjusting its size, Quantum Dot can absorb light at wavelengths of more than 1,000 nm and can theoretically utilize the entire spectrum of sunlight that reaches the ground."
Quantum-Dot solar cells, since the first reported cell efficiency for the technology of 2.7% in 2010, have reached up to 16.6% maximum efficiency. It is a noteworthy improvement that is deemed exciting within the solar industry. However, in order to ensure further efficiency at a similar pace, stabilizing Quantum Dot surface for solar cells is essential, the way QD-display has.
Experts see more challenges with controlling the surface of quantum dots used in solar cells versus displays. Extracting electrical current from QD solar cells is technically much more difficult than retrieving light from QD-display.
According to Professor Jeong, “Quantum Dot surface is unstable, which is why QD display is made of a core-shell structure with a shell on the outside. When the shell is covered, light is able to pass through whereas electricity cannot. That is the limit we face with QD solar cells." Therefore, Quantum Dots for solar cells must be stabilized by either making the core with a stable material or by adding additional materials (ligands) to the surface. Professor Jeong added, "There is no doubt that it’s extremely challenging to get the surface of Quantum Dot stabilized, but once we do achieve it, it would only be a matter of time before we see rapid commercialization of QD solar cells.”